Matter
Ecosystems are made up of matter, which is defined as anything that has mass and takes up space. Matter in an ecosystem consists of the abiotic and biotic nutrients. For example, matter in the coral reef ecosystem includes components such as water, carbon dioxide, nitrogen, oxygen, sea turtles, coral, rocks, and salt. When matter passes through an ecosystem, it is not lost. Instead, matter is recycled over and over again.
It may surprise you, but the water that exists on Earth today is the same water that was on Earth over 65 million years ago when dinosaurs were alive. That means that you may be drinking the same water that the dinosaurs drank. How is this possible? According to the Law of Conservation of Mass, matter cannot be created or destroyed. It can only be changed from one form to another. Let’s take a look at the water cycle (figure 16-2). Through different complex processes, water gets recycled over and over again within the Earth and atmosphere. Water (liquid) from oceans, rivers, and lakes heats up and evaporates (gas). This vapor (gas) cools down, becoming heavy, and “precipitates” from the sky in the form of rain (liquid), snow (solid), or hail (solid). Plants also recycle water through a process called transpiration. Their roots absorb water (liquid) from the soil and the stem draws water to their leaves. The water evaporates (gas) from the surface of the leaves. These parts of the water cycle repeat over and over again. The water may have changed from solid, liquid, and gas; however, the hydrogen and oxygen atoms that made up the water molecule were never created or destroyed. That means that the same hydrogen and oxygen atoms that existed 65 million years ago are still present today.
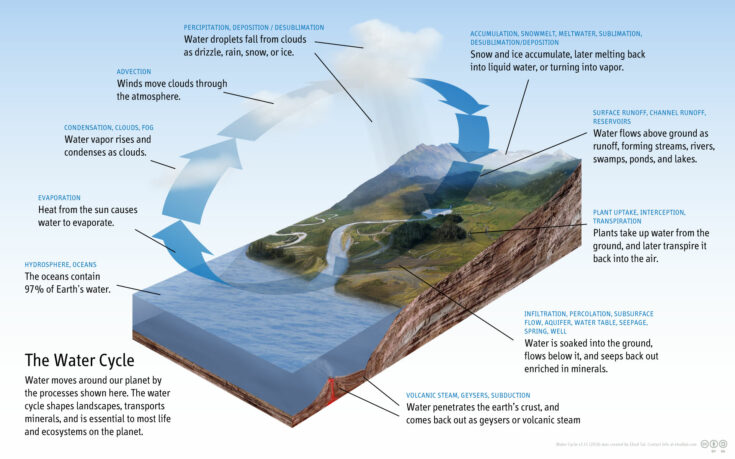
Figure 16-2. Diagram of the water cycle.
Let’s look at another example of how water is conserved during photosynthesis. Figure 16-3 illustrates the molecular and structural formulas that occur during photosynthesis.
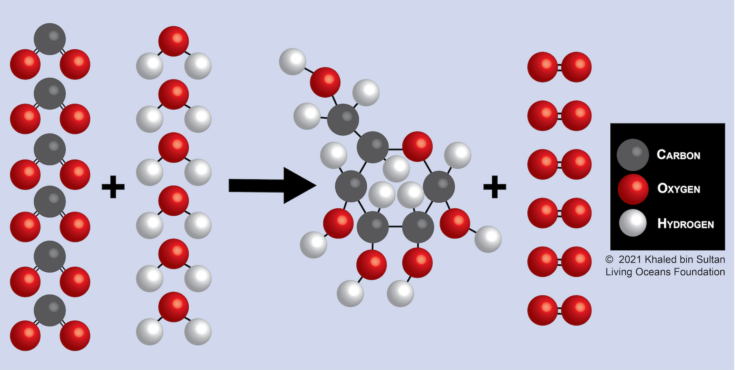
Figure 16-3. Molecular and structural formulas for photosynthesis
Six carbon dioxide molecules (CO2) and six water (H2O) molecules are converted by sunlight (energy) into a sugar molecule (C6H12O6) and six oxygen molecules (O2). Are the same number of atoms present after the chemical reaction takes place?
The same number of atoms are present after the chemical reaction occurs. Even though the original carbon dioxide and water molecules have recombined to form sugar and oxygen (changed from one form to another), the same number of atoms are present. Matter is once again conserved.
Matter is not created or destroyed. Atoms, molecules, and ions are cycled over and over again on Earth. They recombine to create different forms of matter, and some can even change states (solid, liquid, and gas). The matter on Earth today was around during the time of dinosaurs and billions of years before that. Some of the atoms in your body may have come from rocks, water, and even dinosaurs.
In other sections of this unit, you will learn more about how matter cycles through ecosystems.